The road to Eindhoven’s hybrid quantum computer
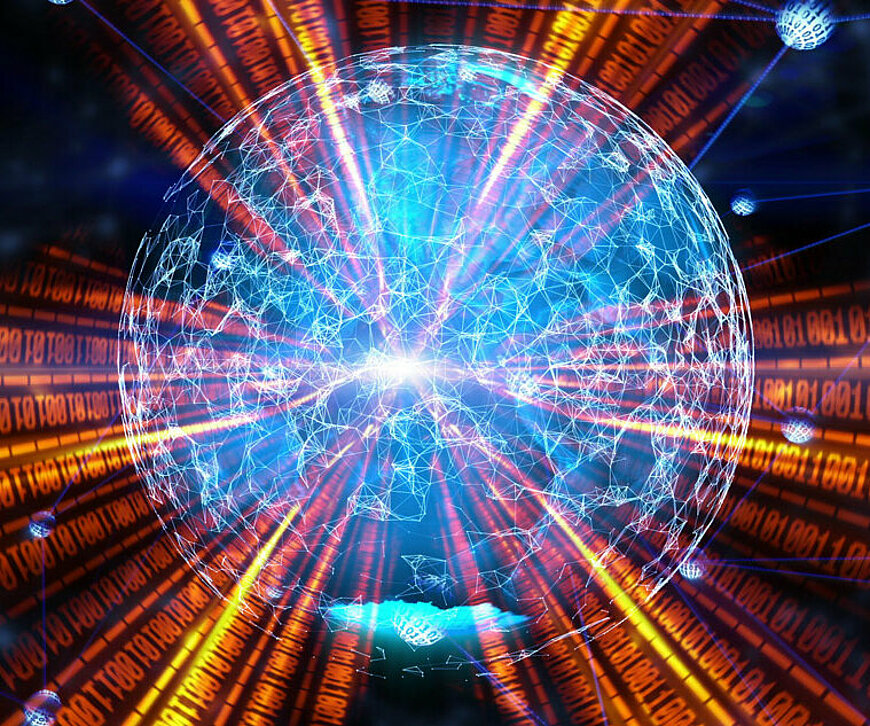
Funding from QuantumDeltaNL and TU/e to help build a hybrid quantum computer on the TU/e campus by 2024.
The need to continue developments in quantum computers is an indicator of the future importance of these computational platforms. In 2020, the Dutch government committed €23.5 million to quantum innovation, an investment coordinated by QuantumDeltaNL. Part of this funding will be used to build a hybrid quantum computer – a device consisting of both classical and quantum computing technologies – at Eindhoven University of Technology by 2024, which will be accessible 24/7 for scientific computations. Physicist Servaas Kokkelmans explains the challenges and quantum enemies that must be overcome to create this unique computer.
Our daily reliance on computers is self-evident. We use computers to send e-mails, order furniture, watch our favorite Marvel films, and to video chat with people around the world.
Computers have also led to once unimaginable possibilities in society. In industry, computers have enhanced and optimized manufacturing processes, created new product lines, and decreased the need for piles of tedious paperwork.
In the world of science and engineering, computers have helped instigate new disciplines such as numerical simulations, bioinformatics, and computational design. Scientific advances in many disciplines would have been impossible without the computer.
Quantum assistance
Nonetheless, even the current generation of supercomputers face insurmountable hurdles in solving problems in certain disciplines such as medicine, quantum chemistry, and material science.
Fortunately, the quantum computing age is gaining significant traction, with researchers such as Associate Professor Servaas Kokkelmans, director and one of the main proponents of quantum technologies at QT/e – the Center for Quantum Materials and Technology Eindhoven – which is TU/e’s dedicated research center for quantum technologies, working on quantum technologies.
He is working towards a future where quantum computers can have a similar impact on society as the classical computer did in the past. “Quantum researchers all want to push quantum technology, and at Eindhoven, we are striving to create a very unique interpretation of the quantum computer – a hybrid quantum computer – a computer that combines classical and quantum computing technologies”, says Kokkelmans. “We’ve cracked the classical part of this problem, but the quantum part still needs work.”
Building a successful quantum computer is fraught with challenges. First, a design must be envisaged, evaluated, and revised. Once a quantum computer design is theoretically confirmed the next step is actually building it. This requires particular materials that must be stored under specific conditions, and as you’ll see this can be quite problematic.
But before delving into the challenges associated with building quantum computers, let’s meet the fundamental component of a quantum computer – the qubit.
Replacing "1 or 0" with "1 and 0"
Classical computers typically store information as bits that can have one of two values – 1 or 0. In other words, they store information in binary format. Every day, you change the information in the bits of computer memory, microprocessors, graphics cards, and magnetic storage devices. Your life really is just the sum of changing bits.
In quantum computers, the classical bits are replaced by quantum bits or qubits. Examples of qubits include photons (with horizontal or vertical polarization) or electrons (with spin-up or spin-down).
Thanks to the wonders of quantum mechanics, a qubit can store both a 1 and 0 state at the same time, and all other states in between. This counter-intuitive quantum behavior is known as superposition, and it means that a group of qubits can theoretically store all programmable values at the same time.
In magnetic storage devices or semiconductor materials in classical computers, bits are represented as tiny bar magnets or a memory cell consisting of one or more transistors respectively. For magnetic materials, the tiny bar magnets can point up or down, with these states representing 1 or 0.
On the other hand, to make qubits, you need to look to the atomic scale, more specifically the electrons that orbit the nucleus of atoms.
“Atoms are the perfect qubits because they are all the same. In addition, they provide us with access to two definite states – spin-up and spin-down – associated with any electron that orbits the nucleus,” says Kokkelmans. “However, only certain atoms can be used as qubits, and to ensure they remain stable for a hybrid quantum computer, these atoms also need to be ultracold.”
Cooling the right qubits
While all atoms have electrons, only certain atoms are suitable to be used as qubits. With one electron orbiting its nucleus, hydrogen might seem like the perfect qubit. Nothing could be further from the truth. “One reason that hydrogen is not used is that it is really hard to cool and trap, and many people have tried to this in the laboratory,” states Kokkelmans.
Appropriate qubit atoms lie elsewhere on the Periodic Table in the form of rubidium (Rb) and strontium (Sr), which can be found in the first and second column of the Periodic Table respectively. Kokkelmans and his colleagues have a lot of experience in working with rubidium for quantum devices but it’s not the perfect qubit atom. “Rubidium is a heavy atom and easy to cool, but there are issues with fidelity – which is a measure of how close your quantum system is to the state that you want it to be in.”
The alternative is to use strontium atoms. “Strontium atoms are more difficult to handle or cool in comparison to rubidium atoms, but they have better fidelity than rubidium atoms. For this reason, we may seek to change the atom and build our hybrid quantum computer at TU/e using ultracold, strontium atoms,” says Kokkelmans.
Counter-intuitively, lasers are used to ultracool atoms to temperatures in the microkelvin range, and Kokkelmans has a nice analogy to explain the use of lasers in cooling. “Imagine an atom as a football, and that the laser emits photons that can be seen as small ping-pong balls. When we shine lasers from a number of different directions on an atom, the photons hit the atom from all directions. As a result, the atom is slowed down in all directions and then confined to an ultracold optical trap.”
Quantum dechoerence - the enemy of the quantum computer
In addition to cooling, lasers are also used to excite atoms to so-called Rydberg states, which is needed to promote interactions between neighboring qubits.
In a crystalline solid, atoms are very close to each other and they can feel or interact with each other all of the time. This arrangement would not work for a collection of qubit atoms, as it would then be almost impossible to isolate the individual atoms using lasers.
As part of their work, Kokkelmans and his collaborators create artificial crystals where the atoms are arranged in a regular lattice using optical tweezers – another term for a focused laser used to hold an atom, but the atoms are much further apart than in a normal crystal. They use a special trick to get the atoms to feel each other. “We excite the atoms to Rydberg states. This allows us to keep the atoms far enough apart so that they are isolated, but as they are excited, that they can still feel each other,” notes Kokkelmans.
The stability of the quantum state of the ultracold qubits can be affected by many factors. External heat or vibrations can perturb the ultracold qubits, while the reliability of the lasers used to hold and manipulate the atoms can have a negative effect. This can lead to quantum decoherence of the qubits, which means that the superposition and entanglement of the qubit quantum states starts to vanish.
“You can look at quantum decoherence as the enemy of the quantum computer, and this is something we really have to worry about,” says Kokkelmans. In principle, it is possible to correct for these effects with quantum error correction, where additional qubits are used to store past quantum states.
However, the thresholds for applying these techniques are quite high, and researchers worldwide are far from implementing them. This is typical of the current era of quantum computing, which is known as the Noisy Intermediate Scale Quantum (NISQ) regime. This refers to qubit devices subjected to decoherence without error correction that are on the threshold of demonstrating quantum speedup with respect to classical computers.
Hybrid quantum computer - best of both worlds
Once a reliable and stable method for storing ultracold strontium atoms is developed and proper single-qubit addressing is included, Kokkelmans and his colleagues from QT/e and QuantumDeltaNL will have their quantum qubit device with high fidelity qubits, although without quantum error correction. They can then take the next step of combining this device with a classical computer to make a hybrid quantum computer.
“A hybrid quantum computer exploits the best of both worlds as it seeks to have the best parts of a quantum device and a classical supercomputer work together,” says Kokkelmans. In a hybrid quantum computer, the quantum device will act as a quantum co-processor that works with a classical device, which will include circuits for control and pre- and post-processing. Moreover, the algorithms that run on a hybrid quantum computer are more robust towards decoherence, and therefore reduce the need for quantum error correction.
But why build a hybrid quantum computer in the first place? Well, it all comes down to application.
“Classical supercomputers are used extensively to do chemical calculations. Perhaps 20% of supercomputer time worldwide is devoted to these calculations. In many cases quantum calculations are required, and that’s where the quantum computer comes to the fore,” says Kokkelmans. “In effect, a hybrid quantum computer could carry out chemical calculations in a more natural and faster manner than a classical supercomputer.”
Plans in place
The plans are very much in place for Eindhoven’s hybrid quantum computer. “We are busy redesigning our setup using qubits based on strontium atoms, and we aim to be fully operational by 2024. Then we will connect our device to the Quantum Inspire network, which means that anyone can run quantum code on the hybrid device,” notes Kokkelmans.
The hybrid quantum computer will be open source and available for anyone to use. People can decide what type of calculation that they want to run on Quantum Inspire and on which quantum platform. For instance, researchers can opt to run chemical or material science calculations on the Eindhoven quantum infrastructure.
While some may anticipate that quantum computers will eventually succeed classical computers, the truth is these computational systems look destined to complement each other in future computational infrastructure such as hybrid quantum computers.
Thanks to research initiatives such as QT/e and their collaboration with the other national research institutes of QuantumDeltaNL, Servaas Kokkelmans and his collaborators look set to be part of the quantum computing revolution.
The challenges and quantum enemies standing in the way of Eindhoven’s hybrid quantum computer look set to be vanquished.